Getting a CRISPR View Into Our Genome
- Julia Colliton
- Mar 12, 2018
- 5 min read

CRISPR-CAS9 (Clustered Regulatory Interspaced Short Palindromic Repeats): Technology that enables scientists to edit parts of the genome.
Humans have always been innovators. Our superior problem solving skills and higher level thinking abilities characterize our species as a people constantly searching for discovery. From the arts to mathematics, the knowledge we have formed is as vast as the oceans covering most of our planet (which we as humans learned how to cross with ease). However, within this immense topic of discovery one domain stands out: science. Not to say scientific discoveries are any more important than Pascal’s triangle or cubism, but science offers a field completely dedicated to research, and there is always something to find.
Recently, the framework of biological research has taken a shift toward genetic research. This shift in it of itself deserves some recognition. We started with a human being as we see it with our eyes. We broke the body into different organ and structural systems. We broke those systems down into cells. We broke those cells down into proteins. We broke proteins into single nucleotides. We went from how the world sees you and me to a phosphate group, a nitrogenous base and a sugar. Those parts form DNA, which we have found to hold our genetic information. This discovery opened more doors than it closed. From the genome project to the field of specific gene editing, advances in this research allows us to map the millions upon millions of nucleotides in DNA, which make up each of our genes. Although we know how to read our genes, we still do not know the specific roles of many genes. This has made genomic modification appealing to many scientists; if we can turn a gene on/off, we can attribute the result to that specific gene. In this gene revolution, one technique deserves recognition: CRISPR.
CRISPR stands for clustered regularly interspaced short palindromic repeats. Don’t let this long, confusing title scare you; CRISPR merely began as an immune response in bacterial cells. The immune system protects cells against foreign pathogens. Like humans, pathogens carry have a genome, with information stored in DNA or closely related RNA. CRISPR recognizes this foreign genome and cuts it up so that it is unable to survive.1 Feng Zhang and associates from the Massachusetts Institute of Technology harnessed this system to use for a new type of gene editing. With CRISPR and a cutting enzyme (usually Cas), they found you can target a specific part of the DNA and cut it out.1 We can think of CRISPR-Cas as a sort of microscopic scissors. Zhang and associates artificially manipulated this system to be of use to countless scientists.
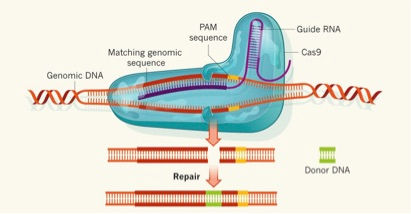
As said before, we care about editing specific genes in order to see their function. Genes are the instructions for the proteins our cells synthesize, and proteins are what make the physical traits we see. The problem we face now is that we have all of the instruction manuals, however they are not labels. Thus, we don’t know how to apply the information we have at our fingertips. Luckily, in science, problems are not bad. They are actually critical; they serve as the starting point for research endeavors. In 2015, Marc Zuckermann and associates used CRISPR-Cas9 in the brains of newborn mice, targeting tumor suppressor genes. 2 They used the microscopic scissors (CRISPR) to cut out these genes and the result was development of glioblastomas and medulloblastomas (tumors) in the mice. By cutting out (or knocking down) these genes, there was an observable, direct correlation between these genes and tumor development. It may seem obvious that these genes hold the instructions to prevent tumors (they are called tumor suppressor genes for a reason), but this study revealed the exact type of tumor that these genes influence. This is the goal for genetic research, not to just have a general idea of what certain genes are associated with, but to actually match each individual gene with the exact mechanism it influences.
Understanding the direct functions of genes provides immense insight into our normal development, but it also holds vast potential into human disease. Disease has plagued humanity from our beginning. Much of medical research throughout history went into controlling communicable disease, to prevent epidemics which led to mass casualty. Now, in developed countries, humans are not as subject to communicable disease as non-communicable. Health in the developed world is more threatened by chronic conditions, such as cancer and autoimmune disease. Many of these condition can be traced back to cells acting haywire. Once again, genetic research comes into play. As Marc Zuckermann knocked down genes that resulted in cancer, many other labs follow suit, in this reverse genetic approach. By artificially manipulating the genome, we can see what goes wrong to cause these illnesses. While this approach holds immense possibility, unfortunately, a large part of the ability for a research lab to make progress comes from adequate funding. Thus, politics becomes a large part in what receives funding. A victim who sees the short end of this deal is Sickle Cell Anemia. This disease is characterized by a mutation in a gene responsible for the production of beta globin, a part of our blood cell which is responsible for delivering oxygen to different organs in the body.3 An individual with Sickle Cell Anemia has misshapen red blood cells, which leads to the blood cells getting clogged in various locations. Victims of this disease often experience severe pain. Sickle Cell was the first genetic disease in which the specific mutation was known, and 60 years later there is still no adequate treatment for it. There is only one drug approved for treating Sickle Cell Anemia and it only helps about half of the patients that receive it. There are fewer than 12 laboratories working on this disease in the United States. As journalist Sharon Begley investigated this discrepancy, she found researchers saying the ‘wrong’ people are affected with this disease. Sickle Cell Anemia isn’t a ‘sexy’ disease, thus it does not get priority in research funds.4 Although this is a pretty striking example, many research labs deal with the same issue of inadequate funding. However, CRISPR sheds a light at the end of this dark tunnel. CRISPR is a vastly easier method of gene editing than previous methods, which could make research into disease more accessible. Many research labs are moving in the direction of CRISPR, which will lead to discoveries that could help millions of people. With more laboratories able to conduct research, there is hope for the many victims of disease.
Knowing the exact function of each gene in the genome would definitely be an instrumental achievement for humanity. However, with this immense knowledge we have to assume responsibility. If we know what each gene does, and we have the ability to manipulate genes, we would have the option to change the genome to produce desired results. Where will this modification end? Will this modification evolve into enhancement? This idea brings up the debate of designer babies. The option to choose characteristics in your child already exists to a point. With genetic screening, people can choose whether or not to have a child if there is a large risk for passing on a genetic disease. But, what if people could choose whether or not their child had blue eyes? Or was a good baseball player? Or had a high IQ? All these questions frighten people when they hear about genome modification, as it could lead to a gap in society between the enhanced and the normals.
With such a strong link between our health and our genomes, it would be detrimental to discount CRISPR due to fear. CRISPR is an emerging tool, with possibilities of vast discovery. With further use, we will need to develop more regulation to address the concerns of the population. The possibilities of CRISPR research are endless, and if we are able to harness its potential, the results will be amazing.
Comments